James E. David and Charles Byvik
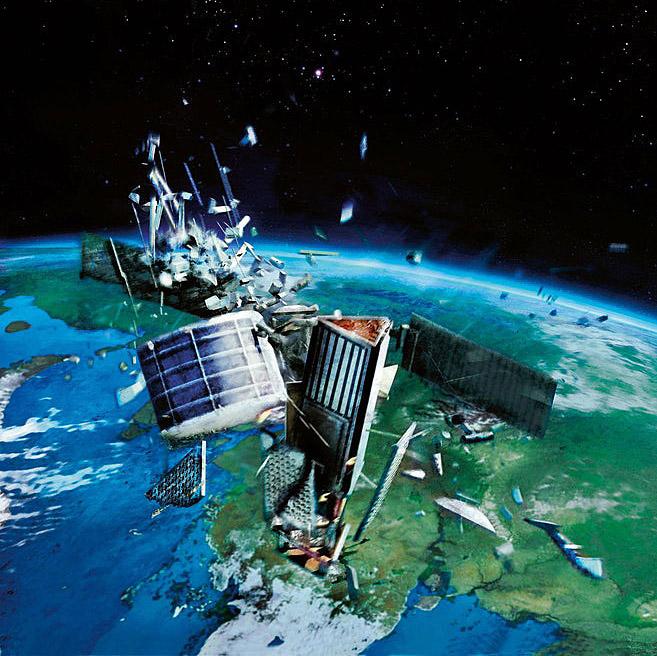
Washington, D.C., March 13, 2023 – The 65-year U.S. effort to detect and track objects in space, from the days before Sputnik 1 to today’s much more crowded orbital environment, is the subject of a fascinating new article and briefing book posted today by the National Security Archive.
Obtained through Mandatory Declassification Review (MDR) requests and archival research, these 25 documents reveal how U.S. policymakers conceived and built the U.S. Space Surveillance Network to detect, track and collect information on an increasingly busy and complex array of satellites and debris.
Compiled and annotated by James E. David, curator of national security space programs at the Smithsonian National Air & Space Museum, and Charles Byvik, the documents trace the origins and evolution of the program, including the development, beginning in 1956, of the first cameras specifically designed to photograph and track U.S. scientific satellites. Other documents detail how in subsequent years the development of the Space Detection and Tracking System (SPADATS) greatly expanded the array of radars and optical sensors to keep up with the ever-increasing number of space objects, especially Soviet military satellites, which represented a potential threat to national security.
Today’s posting draws from a variety of declassified sources, including early CIA memos on requirements for “surveilling” foreign space vehicles, Pentagon reports on various Soviet satellite launches, and the 1965 report of a high-level working group established to review the entire U.S. space surveillance program.
The mission of the Department of Defense’s Space Surveillance Network is to detect, track, and identify all artificial objects orbiting the Earth and provide data on them to a range of users. Ground-based radars and telescopes, augmented in recent years by telescopes on a small number of satellites, acquire the raw data, which is then analyzed to determine specific information on each object detected and tracked. This information is used for a variety of purposes: to maintain unclassified and classified catalogs of all known space objects (U.S. and foreign active satellites and space debris); for Space Object Identification to determine the size, shape, and motion of satellites to assist in determining their missions; to support the targeting of anti-satellite weapons systems; to determine the arrival time of satellites over a certain point on Earth; to predict the decay of objects that enter into the Earth’s atmosphere and their impact point if they survive reentry; and to predict collisions of objects in space.[1] Although there are many gaps in the network’s history due to continuing classification, this briefing book describes the evolution of the Space Surveillance Network from the Sputnik era to the present, based on the available declassified materials and other sources.
1957-1970
The first U.S. assets specifically developed to conduct space surveillance were the large Baker-Nunn cameras built by the Smithsonian Astrophysical Observatory (SAO) to track and photograph U.S. scientific satellites scheduled for launch during the International Geophysical Year from July 1957 through the end of 1958. The SAO oversaw the building, deployment, and operation of these cameras at 12 fixed sites worldwide beginning in 1958 and, starting in 1957, the establishment of Moonwatch teams of amateur astronomers using small telescopes in the United States and abroad. Using information from the Moonwatch teams and other sources to determine where to point their cameras, the Baker-Nunn equipment could photograph satellites up to altitudes of 2,000 miles for a limited number of inclinations. Additionally, two worldwide networks of ground stations—with radars, antennas, and other equipment to track, receive telemetry from, and send commands to, U.S. scientific satellites—were established: the Naval Research Laboratory’s (NRL) Minitrack network for Vanguard satellites and the Jet Propulsion Laboratory’s Microlock network for Explorer satellites.[2]
The performance of these and other assets was first tested when the Soviets launched Sputnik 1 into a low-Earth orbit (an altitude below 1,200 miles) on October 4, 1957. Moonwatch teams quickly obtained information on the orbit that enabled the NRL’s radio array in northern Virginia to acquire the satellite’s continuously transmitted signals beginning on its third orbit to help refine the orbital parameters. Tracking by these passive systems was augmented by several existing operational U.S. radar facilities around the world such as the Air Force radar at Diyarbakir, Turkey, used to detect and track Soviet missiles launched from Kapustin Yar, and selected radars at the Atlantic Missile Range. The Millstone Hill radar under development for the planned Ballistic Missile Early Warning System (BMEWS) by Lincoln Laboratory in Massachusetts was the first U.S. radar to both detect and track Sputnik 1.
Both the Army’s Vanguard Computing Center and the Air Force’s Air Research and Development Command, under Project Harvest Moon, processed data collected by passive and active sensors at these civilian and military facilities to accurately predict current and future orbits. These detection, tracking, and processing assets were quickly exercised again in November with the launch of Sputnik 2 into the same orbit, the world’s second successful satellite. They monitored the new satellite until it reentered the Earth’s atmosphere in early 1958.
Improvements in the collection and processing of data were made during the remainder of the decade that addressed some deficiencies. In 1958, the NRL began construction of the first station of the Naval Space Surveillance (NAVSPASUR) system. Completed early the next year, it had three transmitter and six receiver sites across the southern United States on the 33rd parallel and a control and computational center in Virginia. NAVSPASUR continuously transmitted signals straight up into space and could initially detect objects passing through the signals up to an altitude of 2,000 miles. The Air Force moved the Harvest Moon project to a new National Space Surveillance Control Center in 1958 and began expanding the capabilities for processing data. The next year, it initiated SPACETRACK, a program to deploy improved optical and radar sensors. One of the early actions taken was the acquisition of several Baker-Nunn cameras, the first of which was deployed in Norway in 1960.[3]
The USSR launched more than 150 spacecraft in the following eight years, including photoreconnaissance satellites and spacecraft carrying cosmonauts beginning in 1961, weather satellites starting in 1964, and communication satellites beginning in 1965. Except for communications satellites placed in highly elliptical orbits—also called Molniya orbits—and probes exploring the Moon or planets, all were in low-Earth orbit.[4]
The aggressive Soviet space program clearly presented a potential threat to U.S. national security. The shortcomings of the fragmented DoD space surveillance efforts for global coverage and timely response were addressed in November 1960 when Secretary of Defense Thomas S. Gates, Jr., assigned operational control of the newly designated Space Detection and Tracking System (SPADATS) to the North American Air Defense Command (NORAD). SPADATS included NAVSPASUR, SPACETRACK, and the National Space Surveillance Control Center (which would soon be renamed the SPADATS Center). NORAD quickly took steps to improve the collection and processing of data, such as procuring advanced computers for the SPADATS Center, integrating BMEWS and SPADATS, and initiating the design of a dedicated space surveillance radar for deployment at Eglin Air Force Base in Florida.[5]
NORAD and NASA entered into an agreement in 1961 that detailed their respective responsibilities regarding space surveillance. Based on data from its own and other sensors, NORAD was to include information on all known objects in orbit in a classified space catalog, while NASA was to provide NORAD with all the tracking data its networks acquired on U.S. and foreign spacecraft. NASA would receive all unclassified information on U.S. and foreign spacecraft from NORAD and disseminate it publicly in a separate, unclassified, space catalog. NORAD would provide NASA with classified information when the latter demonstrated a “need to know.”[6]
NORAD published an updated set of space surveillance requirements in early 1965 after extensive consultation with users of its data. The high-level Ad Hoc Working Group on Department of Defense Space Detection, Surveillance, Tracking and Data Processing, established by the Deputy Secretary of Defense the prior year, quickly reviewed the requirements as part of its comprehensive examination of the space surveillance program. Among other things, its lengthy March 1965 report gave a detailed analysis of the capabilities of SPADATS and other military and civilian sensors, as well as SPADATS computer processing and communications facilities. SPADATS had no dedicated radars yet and relied on the three BMEWS radars (in Alaska, Greenland, and the United Kingdom), the missile detection/space surveillance radar on Shemya Island in the Aleutians, the RCA radar at Moorestown, New Jersey, the Millstone radar in Massachusetts, and the Diyarbakir radar in Turkey. All these facilities conducted space surveillance as a secondary mission and had varying capabilities to detect objects, but between them they detected, within the first few orbits, most Soviet satellites and other objects up to altitudes of 2,900 miles that were at least one square meter in size and orbiting at inclinations of 45 degrees and higher. These radars and the SPADATS optical sensors provided a good tracking ability and could predict an orbit after 12 hours of tracking with an accuracy of roughly one mile. The radars and optical sensors also obtained good Space Object Identification information. NAVSPASUR detected within the first five or six orbits most Soviet satellites and other objects up to altitudes of 4,000 miles that were at least one square meter in size and orbiting at inclinations of 30 degrees and higher.[7]
With respect to other military and civilian sensors, a major contributor in several areas was what the report designated the “Intelligence Network.” Although not described further because of higher classification, the “Intelligence Network” referred to the National Security Agency’s worldwide network of ground stations, aircraft, and ships, and Central Intelligence Agency ground stations in several countries, that intercepted telemetry from foreign missiles and spacecraft. Telemetry from these vehicles to ground stations contains information on vehicle performance or data the spacecraft is acquiring, such as photography from weather satellites. It also includes commands from ground stations to these vehicles. The intelligence obtained from the collection and analysis of telemetry was critical to determining the missions of foreign satellites. Certain radars of the “Intelligence Network” and its communications intercept facilities (which monitored launch complex and downrange tracking station traffic) provided alerts on impending launches and enabled faster detection of any payloads that achieved orbit. Lastly, the “Intelligence Network” acquired limited tracking information. Tracking data on DoD satellites came from the Satellite Control Facility and on NASA satellites from NASA networks. Additionally, the Royal Canadian Air Force Satellite Tracking Unit had several sensors that provided information.[8]
The Ad Hoc Working Group also assessed the extent to which SPADATS met user needs. It predicted the arrival of satellites at a given position to better than plus or minus 15 seconds, which was acceptable to most users. SPADATS provided needed support to many military and NASA space programs, including locating malfunctioning objects, predicting the decay of objects of particular interest (e.g., Soviet satellites and rocket stages), and tracking U.S. reconnaissance satellites to assist the Satellite Control Facility. The ability to determine the orbital elements of Soviet satellites and obtain their radar signatures (from which estimates of their size, shape, stability, and orientation could be made) were important contributors to Space Object Identification. Precision tracking information provided by SPADATS was sufficient for the two operational anti-satellite systems (the Air Force’s nuclear-armed Thor missiles on Johnson Atoll and the Army’s nuclear-armed Nike-Zeus missiles on Kwajalein Atoll) to be able to intercept a limited number of spacecraft in low-Earth orbit.[9]
Although the Ad Hoc Working Group agreed with many of NORAD’s new requirements, it recommended disapproval based on technology availability, realistic future needs, and costs. Most significantly, the Working Group believed there was no justification for the technological improvements envisioned by 1970 (to extend altitude coverage to 23,000 miles, to increase the detection capability to objects 0.1 square meter in size without regard to altitude, and to improve the detection probability to 95 per cent for all objects before completion of their first orbit without regard to inclinations or altitude). The Deputy Secretary of Defense approved the Ad Hoc Working Group’s report and directed the Joint Chiefs of Staff to have NORAD revise the requirements to conform with it. The new set was approved by the Joint Chiefs of Staff and the Office of the Secretary of Defense in 1966.[10]
One of the major challenges SPADATS faced was the rising number of spacecraft and the increasing amount of debris in orbit. From January 1967 to June 1969, for example, the USSR launched almost 200 spacecraft and the United States about 85. Almost all were in low-Earth orbit, except for probes, human spaceflight missions to the Moon, Soviet communications satellites, U.S. communications satellites in geosynchronous orbit (22,236 miles above the equator), and U.S. early warning satellites in medium-Earth orbit (an altitude between 1,200 and 22,236 miles). The number of publicly reported tracked objects increased from around 100 in 1961 to over 2,000 by the end of the decade. Although posing little threat to active satellites, there were increasing amounts of space debris in low-Earth orbit, and more of the debris in the lower regions of that orbit (up to 300 miles altitude) was surviving reentry and impacting on land or in the oceans, generating growing public concern about the possibility of damage or injury caused by objects falling from space. (Debris in the upper regions of low-Earth orbit remains in space for hundreds of years, while debris in medium-Earth and geosynchronous orbits can remain there for thousands of years.)[11]
U.S. intelligence agencies were eager to collect the debris left behind by Soviet rockets, missiles, and satellites for analysis. Similarly, they wished to keep U.S. debris (particularly from missiles and classified U.S. satellites) out of Soviet hands to prevent their exploitation. To enable operational commands to take passive defensive action against Soviet photoreconnaissance satellites (such as moving their forces or using camouflage), the Defense Intelligence Agency and the Air Force’s Foreign Technology Division established the Satellite Reconnaissance Advanced Notice Program in late 1965. The system provided data to commands on the orbital elements, swath, and resolution of the satellites, and information on how to predict when they would be overhead. Testing of Soviet offensive space weapons began in 1966 with a fractional orbital bombardment system, a missile-launched nuclear weapons delivery system that flew a sub-orbital path. This system became operational in 1968 but was decommissioned in the early 1980s. The USSR also first successfully tested a co-orbital anti-satellite weapon in 1967. These exploded within 60 feet of the target satellite, releasing shrapnel to destroy it.[12]
SPADATS increased its capabilities in several areas during the remainder of the decade. The SPADATS Center moved to the command’s new underground facility at Cheyenne Mountain in Colorado, procuring improved computer capability and upgrading communications with the sensor sites. Modifications to NAVSPASUR in 1966 increased the detection coverage to 6,000 miles, more than double any other SPADATS sensor. The first phased-array (electronic-steered) space surveillance radar, the AN/FPS-85, began operations at Eglin Air Force Base in Florida in 1969. It was the first sensor with the capability to provide detection and tracking coverage above 6,000 miles, extend surveillance to objects with orbital inclinations below 30 degrees, and simultaneously track multiple objects (200 known objects or 20 unknown objects). The AN/FPS-85 soon was able to identify numerous unknown objects which had been detected but not tracked. The ARPA Lincoln C-Band Observables Radar on Kwajalein entered service in 1969 to image objects in support of the Space Object Identification mission. Two Baker-Nunn cameras were moved to other nations, and all received upgrades that reduced from 24 to 12 hours the amount of time needed to search, locate, and produce an accurate observation of an object, and report the data to the Space Defense Center.[13]
1970-2000
The orbital environment became increasingly crowded and complex between 1970 and 2000. Soviet reliance on satellites to support military operations rapidly increased, and, by 1985, the intelligence community estimated that the Soviet Union had about 140 such satellites in orbit. Because some were now in medium-Earth orbit (such as the GLONASS navigation satellites launched beginning in the 1980s) or geosynchronous orbit (including the early warning satellites launched starting the same decade), it was critical that SPADATS improve its deep space capabilities. More than 30 nations joined the United States, USSR/Russia, United Kingdom, France, Italy, West Germany, and the European Space Agency in becoming owner/operators of satellites. Spacecraft were performing new missions such as remote sensing and navigation. Initiating the commercial development of remote sensing spacecraft, Digital Globe launched Ikonos in 1999. Reflecting both the growth in active satellites and space debris in orbit and the increased detection capabilities of sensors, by 2000 there were nearly 9,000 objects publicly reported as being tracked.[14]
The Soviets declared their co-orbital anti-satellite weapon as operational in the early 1970s, and testing of the system continued until 1982. It was tested successfully against targets at altitudes up to approximately 1,000 miles and inclinations between 40 and 140 degrees. There is evidence that the USSR was developing another system designed to destroy satellites in medium-Earth and geosynchronous orbits, but Russia abandoned the program in the early 1990s.[15]
Major improvements to SPADATS took place during this period, although most details regarding sensor coverage, the improvements in detectability and tracking, and other important aspects remain classified. Defense Support Program early warning satellites entered into service in 1971, augmenting existing sources for launch detection. These satellites reduced the time needed to determine whether a launch was a missile test or space launch from 20-30 minutes to only several minutes. Three years later, the former Safeguard anti-ballistic missile system radar in North Dakota was modified to provide space surveillance information as a secondary mission to its new primary mission of detecting and tracking intercontinental and submarine-launched ballistic missiles in flight. A new phased-array radar, designated Cobra Dane, began operating at Shemya in 1977, increasing the number of objects that could be tracked simultaneously and extending the detection and tracking range. Lincoln Laboratory’s Haystack radar was modified to begin conducting space surveillance the same year. The missile-tracking ARPA Long-Range Tracking and Instrumentation Radar on Kwajalein underwent upgrades in 1982 to enable it to track objects up to geosynchronous altitudes as a secondary mission. New phased-array radars were built at the three BMEWS sites beginning in 1987. In the 1990s, Lincoln Laboratory built the Haystack Auxiliary Radar near Millstone in Massachusetts to collect images of satellites.[16]
Telescopes from the Maui Optical Tracking and Identification Facility entered service the following year to provide both tracking and Space Object Identification data on objects at altitudes of about 3,400 miles and higher. Ground-based electro-optical deep space surveillance (GEODSS) systems in New Mexico, Maui, Diego Garcia, and South Korea, with low-light-level TV cameras, computers, and large telescopes, began operations in the 1980s to track objects from approximately 6,000 miles to 28,000 miles (the South Korean site closed in the early 1990s).[17]
By 2000, the Eglin Air Force Base radar was the most important in tracking objects in low-Earth orbit (over 40 percent of the total). The three GEODSS sites were the most significant for tracking objects in medium-Earth orbit (33 percent) and in geosynchronous orbit (nearly 44 percent).[18]
The processing of data also underwent improvements. Beginning in 1994, the newly established Space Control Center took major steps to automate many tasks being performed manually. These resulted in a much shorter time for providing orbital information to users. New software was installed to determine current and predicted future orbits more accurately.[19]
2000-Present
U.S. and allied military forces have greatly increased their dependence on satellites for reconnaissance, communications, navigation, weather data, and early warning since the turn of the century. While these systems have always been vital for strategic intelligence, they have also become critical for tactical operations. Individuals, non-governmental organizations, businesses and governments around the world depend heavily on a wide range of satellites for communications, navigation, disaster relief, weather information, environmental and agricultural monitoring, geology, oceanography, and many other applications.
Just as the United States and others are becoming more dependent on space systems, however, the threats to their safety and security are increasing. One of the most critical threats is the growing number and sophistication of anti-satellite weapons, particularly those of China and Russia. The two nations have developed robust space situational awareness systems that are critical in their targeting. (Space surveillance is now commonly referred to as space situational awareness.) In 2021, Gen. David Thompson, the U.S. Space Force’s second-in-command, stated that, “Both China and Russia are regularly attacking U.S. satellites with non-kinetic means, including lasers, radio frequency jammers, and cyberattacks.” Information on most of the attacks remains classified. However, a few have been disclosed such as the February 24, 2022, Russian cyberattack on ViaSat communications satellite ground infrastructure that interrupted service to Ukraine and several European nations. Russia has periodically jammed GPS signals in several areas, including in Ukraine since its 2014 invasion and in northern Norway during NATO exercises there. China and Russia also have operational direct ascent (missile-launched) anti-satellite weapons armed with conventional explosive warheads that can destroy satellites in low-Earth orbit. Each are also conducting rendezvous and proximity tests, in both low-Earth and geosynchronous orbits, in which a satellite closely approaches another. These tests could be for the purpose of developing space situational awareness satellites or for the inspection and servicing of satellites. For example, China’s Shijian-21 satellite docked with and towed one of its defunct satellites out of geosynchronous orbit in 2021. However, many experts believe that the tests are part of the development of co-orbital anti-satellite weapons.[20]
Another threat to the safety and security of satellites is the increasing congestion in space. The number of publicly reported tracked objects has grown from 8,927 in 2000 (2,671 active and inactive satellites, 90 space probes, and 6,096 pieces of debris) to about 47,800 today (7,200 active satellites, 19,600 pieces of debris of known origin, and 21,000 pieces of debris of unknown origin or which cannot be tracked repeatedly). Most of the increase in active satellites is the result of the massive number of small satellites launched to form constellations in low-Earth orbit starting in the 2010s, primarily by private firms. For example, the Starlink constellation of small communications satellites now has over 2,000 spacecraft with several thousand more to be added in the coming years. OneWeb is close to completing its constellation of about 900 small communications satellites. Planet’s constellation has around 200 small Earth-observation satellites. In addition to the tracked debris, there are an additional estimated 600,000 to 900,000 fragments between 5 mm and 10 cm in size, and many hundreds of thousands of pieces smaller than 5 mm in size, that cannot be tracked. Several factors contributed to the increase in space debris: the growing number of pieces (sometimes intact) left over from rocket stages and inactive satellites, the 2007 Chinese direct ascent anti-satellite weapon test, the accidental collision between an Iridium communications satellite and an inactive Russian satellite in 2009, and the 2021 Russian direct ascent anti-satellite weapon test. Several active satellites have been damaged by debris in recent years, and the risks to active civilian, commercial, and government satellites of all nations are increasing.[21]
Beginning in the early 2000s, the U.S. government took several steps to share more space situational awareness data with commercial and foreign entities to lessen the risk of collisions damaging or destroying active satellites and to reduce the amount of debris from them. The Air Force established the Space Track website that provides registered owners or operators with basic orbital data from the unclassified Satellite Catalog. These organizations can also receive planning assistance in fixing certain launch and on-orbit anomalies, emergency conjunction assessment messages (specifying the point and time of the closest approach of their satellite with another tracked object when the chance of a collision exceeds a certain threshold), and review of their proposed maneuver plans to avoid collisions. It also began signing agreements with foreign governments and commercial entities under which they receive more information (apparently including limited data from the classified High Accuracy Catalog when needed) and additional assistance such as deorbit and reentry support. To date, the U.S. Space Force has signed agreements with 30 nations and approximately 120 commercial and academic entities. It will shortly transfer responsibility for sharing space situational awareness data with civilian and commercial operators to the Department of Commerce.[22]
Several nations and international organizations have also adopted guidelines that seek to reduce the future growth of debris. In 2001, the U.S. government issued the Orbital Debris Mitigation Standards (updated in 2022), which are applicable to all U.S. satellite launches and operations. Among other things, the document established rules limiting debris release during normal operations and minimizing the potential for post-mission breakups resulting from stored energy (propellants and batteries). The United Nations General Assembly endorsed the Space Debris Mitigation Guidelines of the Committee on the Peaceful Uses of Outer Space in 2007 that set forth general, non-legally binding rules and encouraged members to use “national mechanisms” to implement them. The European Space Agency, Japan, France, and Russia have since adopted similar guidelines (Russia’s compliance is regarded as mixed). Several commercial entities have tested equipment in space to capture existing debris, but any programs to achieve a significant reduction are many years away.[23]
The Space Surveillance Network’s processing capabilities and sensors have undergone significant improvements. Extensive advancements in the former have resulted primarily in more accurate orbital predictions. A new, classified High Accuracy Catalog has incorporated more precise data since its inception in 1999. The new Globus II radar in Norway, reportedly capable of detecting objects smaller than 10 cm at distances of up to almost 28,000 miles, became operational in the early 2000s. The Space Surveillance Fence, transferred to the Air Force in 2004, was decommissioned nine years later. Its replacement on Kwajalein began operations in 2020 and mostly targets objects in low-Earth orbit. GEODSS received new digital cameras, computer resources and supporting equipment. The new Space Surveillance Telescope was moved to Australia in 2017 and became operational in 2022. As with GEODSS, the primary mission is to monitor objects in medium-Earth and geosynchronous orbits. To overcome the limitations of ground-based optical sensors and to provide better coverage of deep space, a series of satellites with telescopes has been deployed. The Midcourse Space Experiment satellite was the first and operated from 2000 until 2008. It was followed by the Space Based Space Surveillance and Advanced Technology Risk Reduction satellites beginning in 2011 and the Canadian Sapphire satellite in 2014. All are in low-Earth orbit. Six Geosynchronous Space Situational Awareness Program satellites have been launched since 2014. They are in drift orbits below and above geosynchronous orbit to monitor objects in that region.[24]
The challenges to the Space Surveillance Network will undoubtedly grow in the coming years. One will be the detection, tracking and identification of the increasing number of active satellites and debris in orbit. As noted above, Starlink will likely add several thousand additional satellites to its constellation. Amazon’s Project Kuiper plans to establish a constellation of 3,236 small communications satellites in low-Earth orbit from 2023 to 2029. The U.S. Space Force intends to launch between 300 to 500 small communications satellites into low-Earth orbit to form the Transport Layer constellation. China will soon complete the Hongyan constellation of 320 small communications satellites in low-Earth orbit.[25]
Another challenge for the Space Surveillance Network will be monitoring the increasing threats to space systems, such as the development of direct ascent and co-orbital anti-satellite weapons. China and Russia will undoubtedly continue to improve their capabilities to attack U.S. military satellites through these and other means. North Korea and Iran have developed several non-kinetic anti-satellite weapons but not direct ascent or co-orbital ones.[26]
Discussions are ongoing at the national and international levels to strengthen the guidelines now in place to reduce the future growth of debris from the ever-increasing number of launches and satellites. One of the most important forums is the United Nations Committee on the Peaceful Uses of Outer Space. However, major disputes over key issues make the prospect of arriving at an effective agreement unlikely.
No comments:
Post a Comment